Dynamics of key viral attack strategy visible for first time
Visualizing and quantifying frameshifting mechanisms in living cells
Many infectious viruses, from HIV to West Nile, rely on a fundamental biological process called frameshifting to maximize their attack. Long identified as a key mechanism that viruses use to proliferate inside their hosts, the real-time dynamics of frameshifting had never been directly observed, until now.
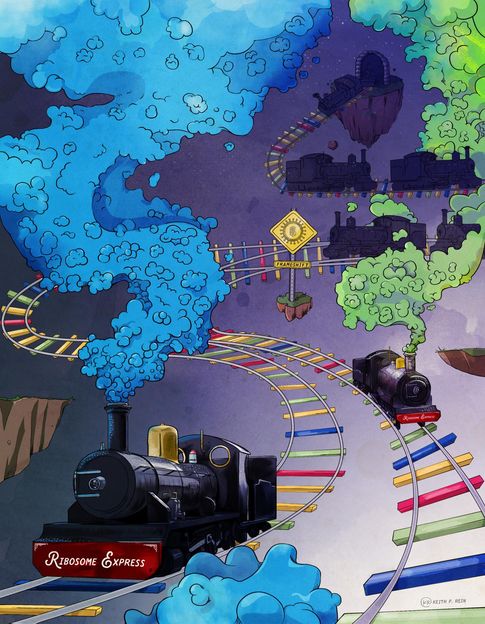
Ribosomes (trains) frameshift (change tracks) at the HIV-1 frameshift sequence (yellow sign), producing large amounts of frameshifted protein (blue smoke) compared to non-frameshifted protein (green smoke).
Keith Rein/Salt & Paper House
For the first time, Colorado State University scientists Tim Stasevich and Brian Munsky have developed detailed imaging technology and computational analyses to visualize, quantify and understand frameshifting mechanisms at the level of single molecules in living cells.
"Our hope is that these integrated experimental and computational methods, allowing us to observe and predict unique aspects of viral replication, can offer strategies for future antiviral therapies," said Stasevich, a Monfort Professor and assistant professor in the Department of Biochemistry and Molecular Biology.
HIV is one example of a retrovirus, meaning it carries genetic information in a molecule called RNA, rather than DNA. When a virus infects a host cell, it makes viral proteins by the manipulation of ribosomes, the cellular protein synthesis machines in which genetic instructions are converted, or translated, from RNA into proteins. During frameshifting, a ribosome that's translating an RNA "slips" one spot backward or forward along a nucleotide sequence, resulting in the translation of an entirely different protein sequence moving forward. This process essentially nets two proteins for the price of one RNA, and it allows viruses to keep their genomes efficiently compact.
Stasevich has been a pioneer in molecular imaging, using engineered protein tags that selectively bind and fluoresce in different colors when, for example, RNA translation occurs. His lab has developed sensitive microscopes that capture these binding events in real time, making movies of heretofore invisible processes. Munsky, a former Richard P. Feynman Fellow at Los Alamos National Laboratory and assistant professor in the Department of Chemical and Biological Engineering, is an expert in building computational models to sift through noisy or "stochastic" single-molecule data to find statistical signatures of hidden biophysical mechanisms
In this latest experiment, Stasevich's team simultaneously monitored the translation of single RNAs into two, unique protein chains during frameshifting, using the HIV-1 virus's binding fragments (not the whole virus).
Peering into this before-hidden world, what the team saw might surprise some biologists.
They found that frameshifting occurs in bursts of activity, after a longer period of non-frameshifting.
It was previously known that, for instance, the HIV virus outputs frameshifted proteins about 5 percent of the time. But instead of lots of RNAs frameshifting at once, the researchers observed a subset of RNAs frameshifting like mad, with about 5 percent of the RNA doing all the frameshifting, as opposed to 100 percent of the RNA sharing frameshifting duties. This demonstrates that frameshifting happens in only a small subset of RNA.
It remains unclear what exactly distinguishes these frameshifting RNA from others, the researchers say. But their newfound ability to target this special subset of viral RNA promises new depth to the understanding of viral replication and could someday inform new antiviral therapeutics.
Munsky's modeling group recreated all the Stasevich lab's frameshifting observations through detailed computer simulations of ribosome traffic along RNA molecules. Aguilera and Munsky showed that temporal fluctuations in the fluorescent data could be reproduced only if ribosomes involved in viral frameshifting sometimes pause at the frameshift site. Their models suggested that these pauses would induce ribosomal "traffic jams" that maintain production of frameshifted proteins long after cessation of regular translation. The models predicted, and imaging experiments validated, that moving the frameshift site to the middle of the RNA induced bigger "traffic jams."
The researchers' next goal is to simultaneously visualize many more - perhaps hundreds - of different RNA and protein molecules, each with their own fingerprint of color, brightness pattern or fluctuation speed. These and other statistical signatures can be visualized and quantified through the team's continued close integration of single-molecule imaging and computational modeling.