Molecules pass through nanotubes at size-dependent speeds
Like a pea going through a straw, tiny molecules can pass through microscopic cylinders known as nanotubes. This could potentially be used to select molecules according to size — for example, to purify water by allowing water molecules to pass through while blocking salt or other substances.
Now, researchers at MIT, Seoul University in Korea and Ursinus College in Pennsylvania have found that such tubes are more selective than had been thought: Molecules of a precise size can zip through five times faster than those that are a bit smaller or larger. The new findings are published in the Nature Communications by MIT professor Michael Strano, graduate students Wonjoon Choi and Zachary Ulissi, and three others.
This size-dependence in nanotube transport was completely unexpected, says Strano, a professor of chemical engineering at MIT. "This work illustrates how transport in pores of this type remains exotic and relatively unexplored," he says.
The team "looked at ion transport through the smallest single nanopores that have been studied," Strano says. The carbon nanotubes they studied had widths ranging from 0.9 to 2 nanometers — about the diameter of a DNA helix — and were about 1 millimeter long.
"What we found was not predicted by theory," he says: Up to a certain diameter, the flow of ions through a nanotube increased steadily — but then beyond that diameter, the flow decreased. "The dependence is a volcano-shaped plot," Strano says.
The peak flow, at the center of that plot, allows transport that is five times greater than transport at smaller or larger diameters. "The experimental results are counterintuitive," Strano says, "that there appears to be an optimal diameter."
This size-dependence of transport could turn out to be useful in a variety of technologies, he suggests, including proton-exchange membrane (PEM) fuel cells, where molecules of oxygen or hydrogen must pass through tiny pores in a membrane to produce electricity. Another potential application is in DNA-sequencing devices, where DNA segments typically hurtle through pores much too quickly to be analyzed. The new understanding may provide a method for "tuning" the transit speed to slow the DNA sequences enough for analysis.
The unexpected size-dependence results from two phenomena, Strano suggests. According to a theory developed by the team, there is first an attractive force, in which ions' electrical charge causes them to be pulled by an electric field through the pore. Since the ions and the tubes are all submerged in water, some water gets pulled along as well.
Up to a certain diameter, those water molecules form a layer, or a few layers, around the ion and are pulled along with it, the team theorizes. But as the opening gets bigger, the water behaves as a bulk material, slowing the ions' passage. "This explanation is consistent with our experimental observations and molecular simulations of water inside of nanotubes of this type," Strano says — though he stresses that while the data on the ion flow is clear-cut, additional theoretical work is needed to fully understand this process.
The finding may help in designing better membranes for desalination of water. The biggest problem with today's membranes is the tradeoff between selectivity versus flow rates: Bigger pores let the water flow through faster, but are less selective. Nanotubes' nonlinear response may provide a way around that.
"The results suggest that by using nanopores of a specific diameter, it may be possible to achieve maximum selectivity with maximum throughput" by optimizing the pore size, Strano says.
The work could also lead to new sensors capable of detecting specific contaminants in water, the team says. For example, arsenic contamination of groundwater is a serious health concern in some regions, but there is no reliable way of testing arsenic concentrations in water. The selectivity of nanotubes might make it possible to design a simple detector that could measure such contamination, Strano says.
Most read news
Other news from the department science
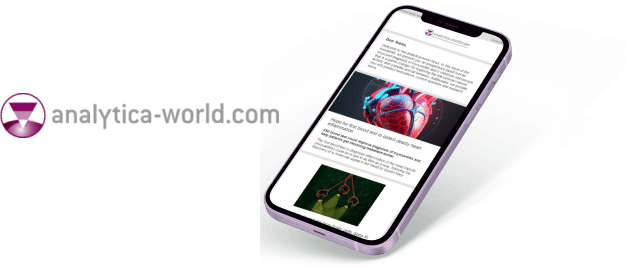
Get the analytics and lab tech industry in your inbox
By submitting this form you agree that LUMITOS AG will send you the newsletter(s) selected above by email. Your data will not be passed on to third parties. Your data will be stored and processed in accordance with our data protection regulations. LUMITOS may contact you by email for the purpose of advertising or market and opinion surveys. You can revoke your consent at any time without giving reasons to LUMITOS AG, Ernst-Augustin-Str. 2, 12489 Berlin, Germany or by e-mail at revoke@lumitos.com with effect for the future. In addition, each email contains a link to unsubscribe from the corresponding newsletter.