Biological specimens imaged with X-rays without damage
Groundbreaking technique using a new kind of focussing lens overcomes previous X-ray imaging limitations
A pollen grain showing the nanofoam within or a diatom with the individual geometric structures inside clearly visible: Using high-energy X-rays from the PETRA III synchrotron light source at DESY, a team led by CFEL scientists Saša Bajt and Henry Chapman has managed to image these structures without damaging them. Their new technique generates high resolution X-ray images of dried biological material that has not been frozen, coated, or otherwise altered beforehand—all with little to no damage to the sample. This method, which is also used for airport baggage scanning, can generate images of the material at nanometre resolution. Using high energy X-rays that are intensely focussed through a set of novel diffractive lenses, the special technique allows imaging to be performed at less than 1% of the X-ray damage threshold of the specimen. The results, which reveal this method as a promising tool for brighter next-generation light sources such as the planned upgrade project PETRA IV, have been published in the journal Light: Science & Applications.
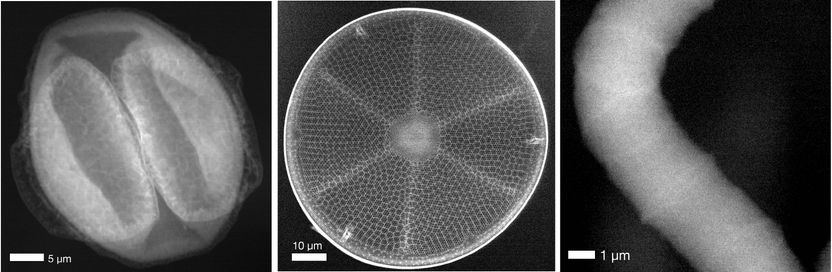
From left to right: a pollen grain collected at DESY, a diatom, and a cyanobacterium, all imaged using Compton X-ray Scattering Microscopy at PETRA III. These micrographs, which reach up to 70 nanometre resolution, could be taken without causing damage to the original samples by using high-energy photons that were highly focussed by novel custom lenses. With the planned PETRA IV light source, such images could achieve even higher detail with finer structural detail, as well as three-dimensional perspectives.
DESY/CFEL
X-ray light interacts with biological material in a variety of ways, mostly depending on the energy and intensity of the light. At the same time, radiation damage, such as small structural changes up to complete degradation of the sample, is the limiting factor during X-ray imaging of biological samples. At low energies, the X-rays are primarily absorbed by the atoms in the sample, whose electrons take on the energy, causing them to spring out of the atoms and cause damage to the sample. Images using these low-energy X-rays thus map out the sample’s absorption of the radiation. At higher energies, absorption is less likely, and a process called elastic scattering occurs, where the X-ray photons “bounce” off of the matter like billiard balls without depositing their energy. Techniques such as crystallography or ptychography use this interaction. Nevertheless, absorption can still occur, meaning damage to the sample happens anyway. But there is a third interaction: Compton scattering, where the X-rays leave only a tiny amount of their energy in the target material. Compton scattering had been largely ignored as a viable method of X-ray microscopy, since it requires even higher X-ray energies where until now no suitable high-resolution lenses existed.
“We used Compton scattering and we figured out that the amount of energy deposited into a sample per number of photons that you can detect is lower than using these other methods,” says Chapman, who is a leading scientist at DESY, a professor at Universität Hamburg, and inventor of different X-ray techniques at synchrotrons and free-electron lasers.
The advantage of low dose in the sample posed a challenge for making suitable lenses. High-energy X-rays pass through all materials and are hardly refracted, or bent, as needed for focussing. Bajt, who is a group leader at CFEL, led efforts to develop a new kind of refractive lens, called multilayer Laue lenses. These new optics comprise over 7300 nanometre-thin alternating layers of silicon carbide and tungsten carbide that the team used to construct a holographic optical element that was thick enough to efficiently focus the X-ray beam.
Using this lens system and the PETRA III beamline P07 at DESY, the team imaged a variety of biological materials by detecting Compton scattering data as the sample was passed through the focused beam. This mode of scanning microscopy requires a very bright source – the brighter, the better – which is focused to a spot that defines the image resolution. PETRA III is one of the synchrotron radiation facilities worldwide which is bright enough at high X-ray energies to be able to acquire images this way in a reasonable time. The technique could reach its full potential with the planned PETRA IV facility.
To test the method, the team used a cyanobacterium, a diatom, and even a pollen grain collected directly outside the lab (“a very local specimen,” Bajt laughs) as their samples, and achieved a resolution of 70 nanometres for each.
Moreover, when compared with images obtained from a similar pollen sample using a conventional coherent-scattering imaging method at an energy of 17 keV, Compton X-ray microscopy achieved a similar resolution with 2000 times lower X-ray dose. “When we re-examined the specimens using a light microscope after the experiment, we could not see any trace of where the beam had come in contact with them,” she explains – meaning no radiation damage was left behind.
“These results could even be better,” Chapman says. “Ideally, an experiment like this would use a spherical detector, because the X-rays coming out of the sample go in every direction from the sample. In that way, it’s a bit like a particle physics collision experiment, where you need to collect data in all directions.”
Additionally, Chapman pointed out that the image of the cyanobacteria is relatively featureless as compared to the others. However, the data indicate that at a higher brightness, such as that of the planned PETRA IV upgrade, individual organelles and even structures in three dimensions would become visible – up to a resolution of 10 nm without damage being a problem. “Really, the only limitation of this technique was not the nature of the technique itself but rather the source, namely its brightness,” says Bajt.
With a brighter source, the method could then be used for imaging whole unsectioned cells or tissue, complementing cryo-electron microscopy and super-resolution optical microscopy, or for tracking nanoparticles within a cell, such as for directly observing drug delivery. The characteristics of Compton scattering makes this method ideal for non-biological uses as well, such as examining the mechanics of battery charging and discharging.
“There hasn’t been anything like this technique in the literature yet,” says Bajt, “so there is much to explore going forward.”
The experiment involved researchers from DESY, CFEL, the Hamburg Centre for Ultrafast Imaging excellence cluster (CUI) of the Universität Hamburg, and Lund University in Sweden.
Original publication
Dose-efficient Scanning Compton X-ray Microscopy; Tang Li, Lukas Dresselhaus, Nikolay Ivanov, Mauro Prasciolu, Holger Fleckenstein, Oleksander Yefanov, Wenhui Zhang, David Pennicard, Ann-Christin Dippel, Olof Gutowski, Pablo Villanueva-Perez, Henry N. Chapman, Saša Bajt; „Light: Science & Applications“, 2023