Record-breaking magnetic shielding for high-precision experiments
The weakest magnetic field in the solar system
magnetic fields easily penetrate matter. Creating a space practically devoid of magnetic fields thus presents a great challenge. An international team of physicists has now developed a shielding that dampens low frequency magnetic fields more than a million-fold. Using this mechanism, they have created a space that boasts the weakest magnetic field of our solar system. The physicists now intend to carry out precision experiments there.
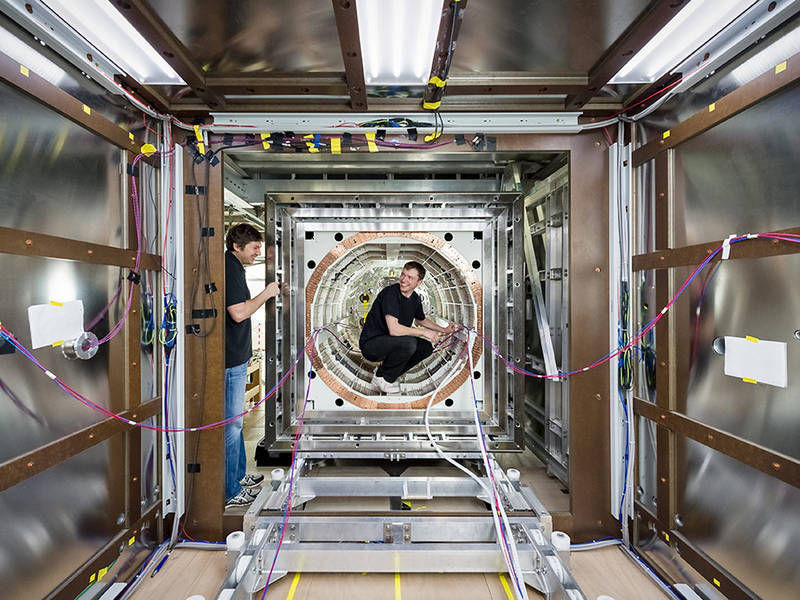
Prof. Peter Fierlinger (l) and co-author Michael Sturm working at the magnetically shielded measuring room.
Astrid Eckert / TUM
Magnetic fields exist everywhere in the universe. Here on the Earth, we are permanently exposed to both natural and artificial magnetic fields. In Central Europe the Earth’s ever-present magnetic field measures 48 microtesla. On top of this come local magnetic fields generated by transformers, motors, cranes, metal doors and the like.
A group of physicists headed by Professor Peter Fierlinger, physicist at the Technische Universität München (TUM) and researcher of the Cluster of Excellence “Origin and Structure of the Universe” have now successfully created 4.1 cubic meter space at the Garching research campus in which permanent and temporally variable magnetic fields are reduced over a million-fold.
This is accomplished using a magnetic shielding comprising various layers of a highly magentizable alloy. The ensuing magnetic attenuation results in a residual magnetic field inside the shield that is even smaller than that in the depths of our solar system. The approach improves the attenuation of previous set-ups more than ten-fold.
Precision experiments on the electric dipole moment of the neutron
Reducing electromagnetic noise is a key prerequisite for many high-precision experiments in physics – but also in biology and medicine. In fundamental physics, the highest degree of magnetic shielding is essential when making precision measurements of miniscule effects in phenomena that drove the early development of our universe.
Peter Fierlinger’s team is currently developing an experiment to determine the charge distribution in neutrons –referred to by physicists as the electric dipole moment. Neutrons are nuclear particles that have a tiny magnetic moment but are electrically neutral. They comprise three quarks, whose charges cancel each other out.
However, scientists suspect that neutrons have a tiny electric dipole moment. Unfortunately, past measurements were not sufficiently precise. The new, nearly magnetic field free space provides the requisite conditions for improving measurements of the electric dipole moment by a factor of 100. This opens the door to a realm of the theoretically predicted scale of the phenomenon.
Physics beyond the limits oft the Standard Model
“This kind of measurement would be of fundamental significance in particle physics and swing wide open the door to physics beyond the Standard Model of particle physics,” explains Peter Fierlinger. The Standard Model describes the characteristics of all known elementary particles to a high degree of precision.
Yet, there are still phenomena that cannot be adequately explained: Gravity, for example, is not even considered in this model. The Standard Model also fails to predict the behavior of particles at very high energies as they prevailed in the early universe. And, it provides no explanation for why matter and antimatter from the Big Bang did not annihilate each other completely, but rather a small amount of matter remained from which we and our surrounding, visible universe are ultimately formed.
Physicists therefore attempt to create short-lived conditions as were prevalent in the early universe using particle accelerators like the Large Hadron Collider (LHC) at CERN. They smash particles into each other at high energies, in particular to create new particles.
Alternatives to high-energy physics
The experiments of the TUM scientists complement those in high-energy physics: “Our high-precision experiments investigate the nature of particles at energy scales that will likely not be reached by current or future generations of particle accelerators,” says doctoral candidate Tobias Lins, who worked on the magnetic shield setup in Peter Fierlinger’s laboratory.
Exotic and hitherto unknown particles could alter the properties of known particles. Thus, even small deviations in particle characteristics could provide evidence for new, previously unknown particles.
In addition to scientists of TU München, physicists of the Physikalisch-Technischen Bundesanstalt Berlin, the University of Illinois at Urbana-Champaign, USA, the University of Michigan, USA, and IMEDCO AG in Switzerland contributed to the experimental setup and measurements of magnetic attenuation. Funding was provided by the German Research Foundation (DFG) in the context of the Priority Program SPP 1491 and the Custer of Excellence Origin and Structure of the Universe.
Original publication
I. Altarev et al.: A large-scale magnetic shield with 10^6 damping at mHz frequencies; Journal of Applied Physics, 12. Mai 2015