Exploring nanoscale objects and processes with microwave microscopy
When lots of energy hits an atom, it can knock off electrons, making the atom extremely chemically reactive and initiating further destruction. That's why radiation is so dangerous. It's also why high-resolution imaging techniques that use energetic electron beams and X-rays can alter, even obliterate, the samples they explore. For example, monitoring battery dynamics using electron microscopy can introduce artifacts that interfere with electrochemical processes. Another case in point: Employing X-ray spectroscopy to see inside a living cell annihilates that cell.
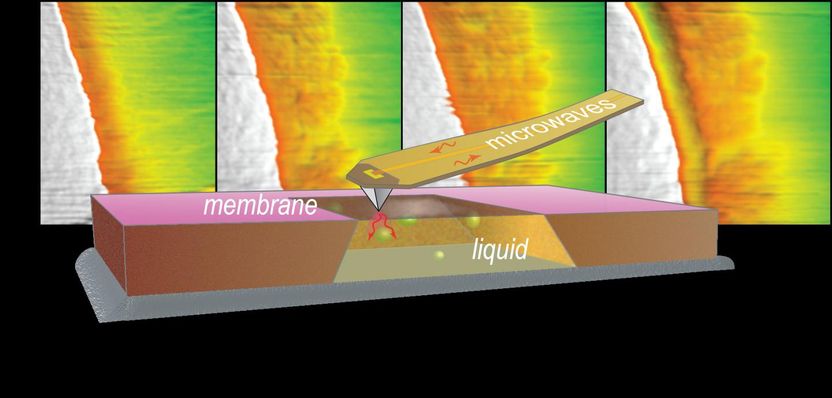
Nanoscale imaging in liquids is critical for understanding key electrochemical processes and the design of rechargeable batteries. A novel approach using a combination of microwaves, a scanning probe and ultrathin membranes avoids the radiation damage caused by imaging methods employing highly energetic X-ray and electron beams.
Oak Ridge National Laboratory, U.S. Dept. of Energy. Image by Alexander Tselev and Andrei Kolmakov
Now, researchers at the Department of Energy's Oak Ridge National Laboratory and the National Institute of Standards and Technology have demonstrated a nondestructive way to observe nanoscale objects and processes in conditions simulating their normal operating environments. They start with an "environmental chamber" to encapsulate a sample in a liquid. The chamber has a window made of an ultrathin membrane (8 to 50 billionths of a meter, or nanometers, thick). The tip of a scanning probe microscope moves across the membrane, injecting microwaves into the chamber. The device records where the microwave signal was transmitted versus impeded and creates a high-resolution map of the sample.
Because the injected microwaves are 100 million times weaker than those of a home microwave oven, and they oscillate in opposite directions several billions of times each second so potentially destructive chemical reactions cannot proceed, the ORNL-NIST technique produces only negligible heat and does not destroy the sample. The scientists report their novel approach of combining ultrathin membranes with microwaves and a scanning probe--called scanning microwave impedance microscopy, or sMIM--in the journal ACS Nano.
"Our imaging is nondestructive and free from the damage frequently caused to samples, such a living cells or electrochemical processes, by imaging with X-ray or electron beams," said first author Alexander Tselev. With colleagues Anton Ievlev and Sergei Kalinin at the Center for Nanophase Materials Sciences, a DOE Office of Science User Facility at ORNL, he performed high-resolution microwave imaging and analysis. "Its spatial resolution is better than what is achievable with optical microscopes for similar in-liquid samples. The paradigm can become instrumental for gaining important insights into electrochemical phenomena, living objects and other nanoscale systems existing in fluids."
For example, microwave microscopy may provide a noninvasive way to explore important surface phenomena occurring on the scale of billionths of a meter, such as the formation of a thin coating that protects and stabilizes a new battery's electrode but cannibalizes its electrolyte to make the coating. Microwave microscopy, which allows scientists to watch processes as they're happening without stopping them cold, makes it possible to characterize ongoing chemical reactions at different stages.
"At NIST, we developed environmental chambers with ultra-thin membranes to perform electron microscopy and other analytical techniques in liquids," said senior author Andrei Kolmakov. He and colleague Jeyavel Velmurugan at NIST's Center for Nanoscale Science and Technology made chambers to enclose objects and processes in liquid environments and performed preliminary characterizations to identify biologically interesting cells. "Conversations between the ORNL and NIST scientists resulted in the idea to try nondestructive microwaves so the environmental chambers could be used for broader studies. There are very few groups in the world that can image with high resolution using microwaves, and CNMS is among them. The design of the experiment and the adjustment of the technology for imaging required ORNL expertise."
The ORNL and NIST researchers combined existing technologies in new ways and came up with a unique approach that may prove useful in medical diagnostics, forensics and materials research.
"For the first time, we are able to image through a very thin membrane," Tselev said. "Microwaves and scanning probe microscopy allowed that."
The right tool for the job
To image highly ordered materials, such as crystals, researchers can employ techniques such as neutron scattering and X-ray diffraction. To image less ordered materials, such as living cell membranes, or processes, such as ongoing chemical reactions, the ORNL-NIST team collaborated closely to innovate the right tool for the job.
Once the scientists had combined the environmental chamber with a scanning microwave capability, they investigated a model system to see if their new technique would work and to set a baseline for future experiments. They used the sMIM system to map polystyrene particles self-assembling into densely packed structures in a liquid.
With that proof-of-principle achieved, they then asked if their system could discriminate between silver, which is an electrical conductor, and silver oxide, an insulator, during electroplating (an electrically induced reaction to deposit silver onto a surface). Optical microscopy and scanning electron microscopy are not good at distinguishing silver from silver oxide. Microwave microscopy, in contrast, unambiguously distinguished insulators from conductors. Next, the researchers needed to know that observation with sMIM would not introduce artifacts, such as silver precipitation, that scanning electron microscopy may cause--a problem that is not trivial. "One paper lists 79 chemical reactions induced by electrons in water," Tselev noted. Generally, scanning electron microscopy will not allow scientists to follow silver precipitation to form growing dendrites because that technique is destructive. "Dendrites behave very badly under an electron beam," Tselev said. With sMIM, electrochemical artifacts and process stoppage did not occur. "Whereas sMIM is not the only nondestructive technique, in many cases it may be the only one which can be used."
Next the researchers imaged living cells. Because healthy and sick cells differ in properties such as the ability to store electrical energy, intracellular mapping could provide a basis for diagnosis. "Tomographic imaging--resolution across the depths--is possible with microwaves as well," Tselev said.
"If you have microwaves, you can go variably in depth and get a lot of information about the living biological cell membrane itself--shape and properties that depend very much on the chemical composition and water content, which in turn depend on whether the cell is healthy or not." The researchers were able to detect properties distinguishing healthy from sick cells.
In the current experiments, the system allowed observation close to surfaces. "That doesn't mean we'll not be able to see deeper if we redesign the experiment," Tselev said. "Microwaves can penetrate very deeply. The depth is basically limited by the contact size between the probe and the environmental cell membrane."
Next the researchers will try to improve the sensitivity and spatial resolution of their system. Because thinning the walls of the environmental chamber would improve the resolution, the researchers will try making the walls with graphene or hexagonal boron nitride, both of which are only one atom thick. They will also use different probes and image-processing algorithms to improve resolution at different depths.